|
|
 |
 |
|
Higgs Force: Seeing the World Through Kaleidoscope Eyes
|
 |
 |
|
Picture yourself in a boat on a river, With tangerine trees and marmalade skies, Somebody calls you, you answer quite slowly, The girl with kaleidoscope eyes.
Lucy in the Sky with Diamonds – John Lennon and Paul McCartney
|
 |
 |
|
Take a quick look around the natural world. We are surrounded by various objects of all shapes and sizes, but there is little sign of pattern or regularity at first glance. We have no obvious reason to believe that all the objects in our vicinity have a similar composition at their most fundamental level and that symmetry is an essential component in the description of their innermost structure. This must be how the world appeared to most observers throughout history. Despite this, around 2,500 years ago, the ancient Greek philosophers believed that they could discern patterns beneath the surface. We do come across symmetrical natural objects from time to time; maybe a pearl in an oyster shell, flowers in a field, a starfish on the beach, snowflakes in a winter storm or a crystal in a rock face. We find their symmetrical shapes intriguing and beautiful. The Greek philosophers believed that these symmetrical shapes hinted at much deeper symmetries at the heart of matter and they believed that numbers and geometry held the key to understanding these patterns. They felt that these exceptional objects might be the key to the fundamental structure of the universe.
|
 |
|
|
 |
 |
 |
|
One of these philosophers Plato attempted to use elegant geometrical ideas to construct what might be considered a subatomic theory of the four elements: Earth, Air, Fire and Water. He suggested that each element is composed of fundamental tiny constituents that have the shape of one of the regular solids, with the fifth solid forming some sort of cosmic essence. We now, of course, know that Plato’s model certainly does not correspond to the true structure of matter. But Plato’s geometrical alchemy had a great influence on later thinkers. In particular, it provided inspiration for the German mathematician and astronomer Johannes Kepler. In 1611, Kepler was invited to the New Year celebrations of a close friend. He later recalled that on one winter’s day:
“.... by a happy chance water-vapour was condensed by the cold into snow, and specks of down fell here and there on my coat, all with six corners and feathered radii. Upon my word, here was something smaller than any drop, yet with a pattern; here was the ideal New Year’s gift, .... the very thing for a mathematician to give, .... since it comes down from heaven and looks like a star.”
Unable to preserve a beautiful symmetrical snowflake as his present, Kepler was inspired to write a little booklet about the snowflake and its symmetry in honour of his friend. This booklet is called ‘De Niva Sexangular’ (The Hexagonal Snowflake). Kepler recognised that although the exact shape of the icy filigree of each snowflake is different, they all display the same hexagonal symmetry. It was the origin of this symmetry that intrigued Kepler. His conclusion was that if materials were really composed of spherical atoms, as some of the ancient philosophers had supposed, then maybe the symmetrical shape of a crystal or a snowflake was the result of the regular arrangement of the atoms from which the crystal was formed.
|
 |
 |
|
The existence of atoms would not become widely accepted for many years. The evidence for them would become much stronger with the realisation that substances had different chemical properties because they were composed of different types of atoms. But, the subject of chemistry was still in its infancy in Kepler’s day. Its evolution from alchemy into a modern science was a long and gradual process. The person whose work was most important in this regard was Antoine Lavoisier who is often regarded as the father of chemistry. Lavoisier realised that while most materials could be chemically decomposed into simpler substances, there were a number of chemicals that appeared to be fundamental in that they could not be broken down any further. These are the chemical elements. Lavoisier formulated a list of 37 such substances. In his treatise The Elements of Chemistry published in 1789 Lavoisier demolished the ancient theory of the four Elements: Earth, Air, Fire and Water, and replaced it with a theory of the chemical elements that we would recognise today. He demonstrated that Water could be decomposed into hydrogen and oxygen. He showed that Air was composed of different gases. And, as for Earth, he demonstrated that there were a number of different solids that he considered to be fundamental ‘earths’. Most of the items on Lavoisier’s list of elements were correct. These include: copper, gold, lead, iron, mercury, silver, tin and zinc, sulphur, phosphorus, oxygen, hydrogen, nitrogen and carbon.
However, Lavoisier’s remarkable scientific research was cut tragically short. During the Reign of Terror that gripped Paris following the French Revolution, Lavoisier was arrested and after a brief trial he was found guilty of treason. On May 9th 1794 he was taken to the Place de la Revolution and guillotined. The mathematician Joseph Louis Lagrange exclaimed sorrowfully,
“It took them only an instant to cut off that head and a hundred years may not produce another like it.”
|
 |
 |
|
The portrait of Dmitri Mendeleyev, gives us the impression of Russian orthodox anchorite, possibly a character from a Dostoyevski novel or even the “mad monk” Rasputin. But Mendeleyev is the world’s most famous chemist and the author of the Periodic Table of the Elements, the key to the whole subject of chemistry. In 1861, Mendeleyev began writing a textbook The Principles of Chemistry that would become a classic, with translations into English, French and German. While planning its outline, Mendeleyev realised that he needed a way to organise the chemical elements that would illustrate the connections between their chemical properties and provide a natural structure for his material. Mendeleyev brought his encyclopaedic knowledge of chemistry to bear on this problem. His solution would change the science of chemistry forever.
|
|
|
 |
 |
|
One early morning, while awaiting a sleigh-ride to the railway station, Mendeleyev picked up a pack of playing cards to pass a few quiet moments playing patience. He shuffled the deck, dealt the cards on his table and began to play. Then, Mendeleyev had a brilliant idea: perhaps the chemical elements could be organised in a similar way to the playing cards. He made a set of blank cards and on each card he wrote the symbol for one of the chemical elements. He then began moving the cards around to see what patterns he could make based on the atomic masses of the elements and their chemical properties. The sleigh arrived to take him to the station, but Mendeleyev continued to search for a pattern. He struggled through the morning playing what he would later refer to as his ‘chemical patience’. He couldn’t quite make sense of the patterns, but he knew that he was very close to an important breakthrough. Eventually he asked his house-keeper to dismiss the sleigh-driver with a request that he return in time for the afternoon train. Finally, frustrated and drowsy Mendeleyev fell asleep in his chair. It was during this brief nap that the final structure of the Periodic Table emerged. In Mendeleyev’s words, “I saw in a dream a table where all the elements fell into place as required. Awakening, I immediately wrote it down on a piece of paper.”
|
 |
 |
|
When the elements were ordered according to their atomic mass, they fell naturally into sequences in which the chemical properties of the elements were repeated. Mendeleyev stacked these sequences into successive rows to form his table, so that each column of the table consisted of a collection of elements with similar properties. He knew that the patterns that he had found must represent important truths about the structure of matter. He was so sure that he was correct that he explained away apparent discrepancies in the table by arguing that some of the published measurements of atomic masses might be incorrect. He also suggested that several gaps in the table could be plugged by predicting the existence of previously unknown elements. Below aluminium, for instance, Mendeleyev’s predicted that there must be a missing element that would fill a gap. He deduced the atomic mass of the missing element and calculated its density, boiling point and the chemical compounds that the element would form. In 1875, a new element closely matching Mendeleyev’s predictions was isolated by a French chemist and named gallium. Over the next decade Mendeleyev scored more successes. One element that he had predicted was found by a Swedish chemist and named scandium. And in 1886, a German chemist confirmed another prediction with the discovery of a new element that he named germanium.
The periodic patterns in the properties of the elements discerned by Mendeleyev is an indication of a deeper layer of order in the structure of matter. The debate about the reality of atoms continued through the 19th century. It was only when the components of the atom, the electrons and the nucleus, were being studied in experiments at the turn of the century that the existence of atoms could no longer be denied. It is the configuration of the electrons in their atoms that determines the chemical properties of each element and this is the ultimate source of the regular patterns in the Periodic Table. This relationship was finally revealed by the pioneers of quantum theory in the 1920s.
|
 |
 |
|
Symmetry is the key to the structure of matter at every level. It is also plays a vital role in modern theories of the forces that hold matter together. But what exactly do we mean by symmetry?
David Brewster was one of the leading British scientists of the 19th century. Throughout his long academic career he invented many ingenious optical instruments including the kaleidoscope, a toy that is just as popular today as it was two hundred years ago. Its only function is to dazzle the eye with an endless sequence of symmetrical images. The kaleidoscope is an enclosed tube containing a number of precisely aligned mirrors. At one end of the tube is a compartment containing coloured glass beads. This end is translucent to allow light into the tube to illuminate the beads. At the other end of the tube there is an aperture through which the eye can peer into the tube. The viewer sees a beautiful symmetrical pattern produced by the multiple reflections of the beads in the mirrors. Twisting the tube shuffles the beads around so that the symmetrical patterns morph into a never ending sequence of new patterns. It is a simple idea, but one with a timeless charm.
It is the arrangement of the mirrors within the kaleidoscope that is the source of its magic. Brewster’s original kaleidoscope contains two rectangular mirrors running lengthways down the tube. The mirrors are joined along one of their long edges and fixed at an angle to each other to give a ‘V’ shaped cross section, with their mirrored sides facing inwards. The angle between the faces of the two mirrors is 30°, or exactly one twelfth of a full rotation. When an assortment of coloured beads is placed between the mirrors, the entire collection undergoes multiple reflections and we see twelve copies of the beads, six of which are mirror reversed. The kaleidoscope produces a hexagonal psychedelic snowflake-like pattern. As the tube is rotated, the beads are rearranged and the pattern changes, but it always retains the hexagonal symmetry.
|
 |
|
|
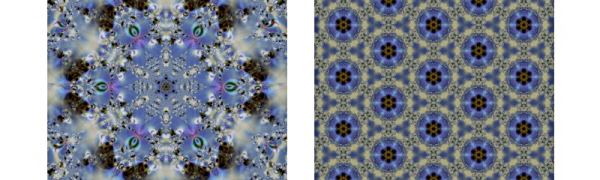 |
 |
 |
|
The illustration on the left above has been generated by constructing a virtual computer model of Brewster’s original kaleidoscope. Multiple reflections in the two mirrors of the virtual kaleidoscope produce a pretty snowflake-like pattern.
Most kaleidoscopes that are found in toy shops today contain three mirrors with a triangular cross section. This gives the kaleidoscopic patterns a higher degree of symmetry, like the illustration shown on the right above. The multiple reflections of the pattern have produced an image that continues in all directions to cover a flat two-dimensional surface.
With three triangular mirrors it is possible to produce a kaleidoscope that will generate all the symmetries of a regular three-dimensional object such as a Platonic solid, such as the image below on the left. Introducing a fourth mirror to form a tetrahedron enables us to generate the symmetries of a pattern that fills three-dimensional space, as shown on the right below.
|
 |
|
|
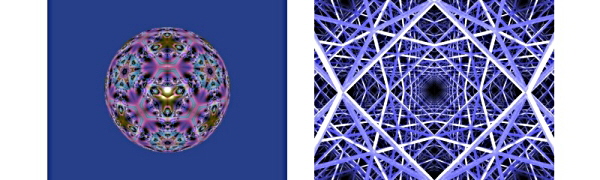 |
 |
 |
|
Symmetry has always been recognised as an important component in art and often when mathematicians and physicists talk about aesthetics in their subjects they are also talking about symmetry. We have an intuitive feeling for what symmetry means. But mathematicians are not content with being able to recognise symmetry when they see it, they require a precise unambiguous definition of what it means. The symmetry of simple figures such as a snowflake or a regular hexagon may be easy to visualise and understand, but a more formal approach is required if mathematicians are to make sense of objects that exhibit more complicated examples of symmetry. The full collection of all the symmetries of an object is called its symmetry group and the area of mathematics that is devoted to the study of symmetry is known as ‘group theory’. It is one of the most elegant and important branches of mathematics. It is studied by pure mathematicians for its intrinsic beauty, but it has also developed into a very powerful mathematical tool that is indispensable for the modern physicist. Fundamental physics has become a search for the most symmetrical theory or laws of the universe.
|
 |
 |
|
In the course of the history of physics, progress has often come about when it has been realised that diverse phenomena have the same root cause. In particular, this has happened when what are apparently different forces have been recognised as just different aspects of a single force. This is described as the unification of the forces of Nature. It is the subject of the next chapter.
|
|
|
|